Next-Generation Protein Delivery via Lipid Nanoparticles
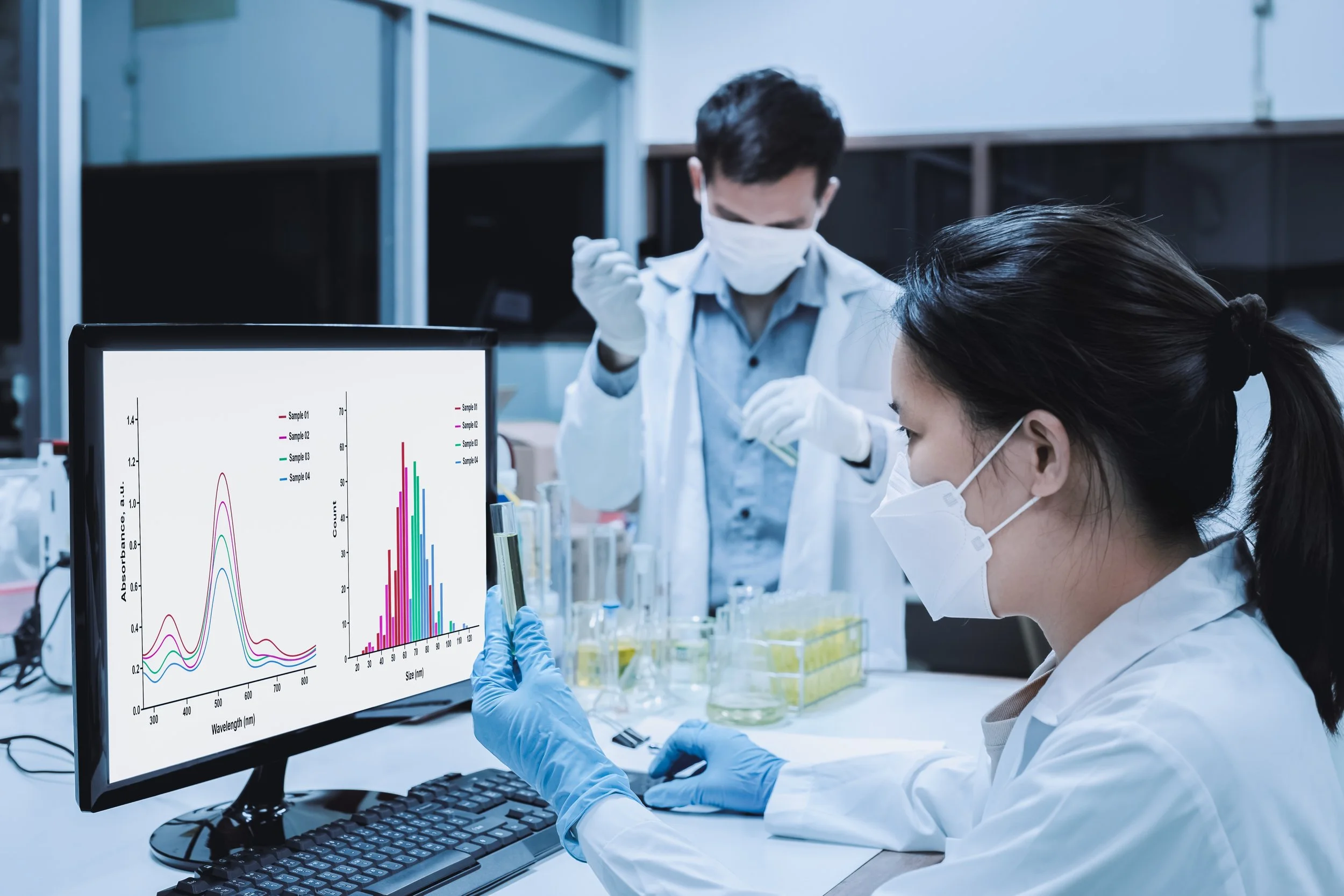
Biomedical research, the ability to deliver therapeutic proteins directly into cells is a pressing need.
This capability can potentially revolutionise treatments for various diseases, enabling precise targeting and reducing side effects. Traditional drug delivery methods, which predominantly rely on small molecules, often fall short when it comes to larger, more complex protein therapeutics.
The challenge lies in effectively transporting these sizeable proteins across cellular membranes to exert their therapeutic effects. Recent advancements in this field have emerged from an interdisciplinary collaboration, promising a novel method to achieve this goal using lipid nanoparticles.
An innovative approach has been developed to "cloak" proteins, allowing them to be encapsulated by lipid nanoparticles, which are comparable to tiny fat bubbles. These nanoparticles can stealthily carry the cloaked proteins into living cells, where they subsequently reveal themselves and perform their intended therapeutic functions. This method could potentially repurpose thousands of commercial protein products, including antibodies, for both research and therapeutic applications.
The details of this groundbreaking technique are outlined in the paper titled "Bioreversible Anionic Cloaking Enables Intracellular Protein Delivery with Ionizable Lipid Nanoparticles," published on May 14 in ACS Central Science. The lead author, doctoral student Azmain Alamgir, conducted this research under the guidance of Chris Alabi, Associate Professor of Chemical and Biomolecular Engineering at Cornell Engineering, and Matt DeLisa, the William L. Lewis Professor of Engineering and Director of the Cornell Institute of Biotechnology.
The inception of this project was driven by a specific objective: to merge the DeLisa group's expertise in designing protein-based therapeutics with the Alabi lab's focus on the intracellular delivery of biologics. For certain drugs to effectively treat diseases, they must penetrate the cell and reach specific intracellular locations, akin to a plumber needing access to a specific room to fix a leak.
Protein-based therapeutics offer significant advantages, including high specificity, lower toxicity, and reduced immune response. However, their delivery into cells poses a substantial challenge due to their large size and complexity, which prevents them from diffusing freely into cells as small molecules do. This limitation has historically made small molecules the primary choice for drug development in the pharmaceutical industry, given their ease of cell penetration without requiring a delivery vehicle.
Over the years, DeLisa's team has created numerous promising protein drug candidates. Yet, their practical application was hampered by the lack of an effective intracellular delivery method. Gene therapy, which can induce therapeutic effects by delivering genes for expression in target cells, was considered. However, this approach has been marred by safety concerns in humans.
"We had been looking for a clever way to efficiently get our engineered proteins inside of cells, especially in a translational context that would not only work in lab-cultured cells, but that would also be effective and safe in animal models and eventually in humans," DeLisa remarked.
The collaboration with Alabi's group provided a new perspective. As DeLisa explained, "When Azmain connected our group with Chris's group, one of the ideas that surfaced was, why deliver this as a gene therapy when we could deliver it as an already-made protein? And so that got us really excited."
Alabi's lab, experienced in delivering nucleic acids into cells using nanoparticles, had struggled with the delivery of "globular squishy soft proteins" due to challenges in producing adequate quantities for testing. "We saw this as a nice bridge between our research groups, to create this new space that I don't think a lot of people were working on at the time and do it in a way that could be scalable and impactful," Alabi noted.
The researchers envisioned a bioconjugation approach to load proteins into lipid nanoparticles, traditionally used for nucleic acids. A significant advantage of this method is its proven success in COVID-19 vaccines developed by Pfizer-BioNTech and Moderna. "At the time, that technology was really taking off," Alamgir recalled.
These vaccines utilised lipid nanoparticles to deliver messenger RNA. The researchers adapted this concept, using lipid nanoparticles to deliver proteins by making them resemble nucleic acids through a "cloaking" process involving a negatively charged ion. This ion allowed the proteins to combine with positively charged lipids through electrostatic attraction.
"The crux of our strategy is conceptually very simple," Alamgir explained. "We're taking proteins and specifically remodelling their surfaces with negative charges, so they look like nucleic acids and can similarly assemble into nanoparticles when formulated with the characteristic lipids."
One challenge was that the formulation conditions for nucleic acids were too harsh for proteins. "We had to use milder conditions and a slightly modified formulation where we added extra lipids," Alabi said. "So both from the protein bioconjugation side, and also from the lipid side, we had to tweak the formulation to make this work as well as it does."
The team, including doctoral student and co-author Souvik Ghosal, demonstrated the cloaking method's effectiveness with lysine-reactive sulfonated compounds, killing cancer cells with ribonuclease A, and inhibiting tumour signalling with monoclonal immunoglobulin G (IgG) antibodies.
A notable benefit of the bioconjugation chemistry is its reversibility. The chemical tag added to the protein detaches once inside the cell's cytoplasm. Given that the method targets lysine, a common amino acid in natural proteins, it can be applied to nearly any protein. "This has potential to take a lot of off-the-shelf proteins that are currently available from many life science distributors and biotechnology companies and repurpose them for novel intracellular applications," Alamgir concluded.
Author:
Alex Carter
Content Producer and Writer